Communities along the U.S. Gulf Coast experience severe flooding events that cause extensive casualties and property damage. In 2005, Hurricane Katrina generated storm surges as high as 11.45 feet (3.5 m) along the state of Louisiana’s coast and cost the country $161 billion in damage, according to the National Oceanic and Atmospheric Administration (NOAA). Robbie Berg at the National Hurricane Center reported that, in 2008, Hurricane Ike inundated the coastal communities of Texas with as much as 10 feet (3.1 m) of water and left damage worth a total of $19.3 billion.
After those and similar flooding events, homeowners may choose to elevate their homes above the base flood elevation, which is a viable mitigation method to reduce or eliminate flood damage. To do this, the existing concrete foundation slabs are typically cast in place directly on the soil support – about 4 inches (102 mm) thick with minimal reinforcement of a single layer of welded wire fabric. The slab perimeter is typically provided with a concrete-grade beam for added stiffness.
The elevation method involves raising the slab home and the attached beams to as much as 15 feet (4.6 m) and placing them on pier supports. For economy and practicality, solid concrete or stacked concrete masonry units are typically used for piers. These are usually placed below the grade beams or newly added steel beams if additional supports are required.
An Existing Knowledge Gap
Elevating a home above the base flood elevation is a viable option for flood damage reduction. However, the elevation process may cause unanticipated deformations and stresses due to the changed support conditions. Such slabs are typically lightly reinforced, and the concrete degrades with age, reducing the capacity and safety. The elevated slabs, therefore, must be properly supported, and inadequacies in these areas can result in possible slab failure, leading to casualties and economic losses. With the proliferation of home elevations in flood-prone areas, the structural safety of such projects is of critical concern.
A knowledge gap exists in the design of elevated home slabs. The American Concrete Institute (ACI) 318-19 Building Code or governing bodies such as the Federal Emergency Management Agency (FEMA) do not provide relevant guidelines or instructions. The former includes guidance on the design of concrete slabs for specific support conditions. However, it does not cover the design of elevated home slabs. The FEMA Homeowner’s Guide to Retrofitting defers such design aspects to trained engineers or contractors. Due to its comparatively low cost and simple design, the current home slab design process has found general acceptance among developers at the expense of an unknown risk of slab cracking, greater deflection, and possible failure. This unknown risk highlights the uncertainty of converting a soil-supported slab into an elevated frame slab without changing the original design.
Furthermore, under the International Residential Code (IRC), which is, by and large, the governing code for residential construction in the U.S., the floor of a single-family residential space must be able to support a minimum of 40 pounds per square foot (psf, or 1.9 kPa) of distributed live load. Any inability of the home floor to support at least that much live load is considered non-conforming according to the IRC provisions. Because of the inherent weakness of the elevated home slab support configuration, several elevated home collapses have been reported to date:
- In 2011, a home in Louisiana collapsed while being lifted as part of the post-Hurricane Katrina relief effort.
- In 2013, after Hurricane Sandy, during elevation, a home in New Jersey slid off its foundation and collided with another as the former was being raised.
- A house in New York also collapsed as it was being raised in the wake of Hurricane Sandy. Many other such examples can be found in the literature.
To address the knowledge gap and safety concerns that exist when elevating a home, the authors conducted a study with two key objectives:
- Examine the safety of elevated home slab configurations and determine the maximum allowed pier spacing to safely support the IRC minimum floor live load.
- Develop a simple tool to check the safety of desired elevated slab configurations that FEMA guidelines or the ACI Building Code do not address.
Experimental Procedure
An experimental study was performed in 2020-2021 to determine how much floor load the typical raised concrete home foundations in residential homes around the Texas Gulf Coast region could safely carry (see Figure 1). Increased floor loads stress the slab to higher levels that can eventually cause the slab to fail through concrete or steel failure. The tests involved pouring water on top of plywood troughs built atop each slab to simulate typical floor loads from everyday use. Data gathered from testing showed how these types of slabs failed and where to expect such failure. It was found that the typical practice of limiting support pier spacing to 10 feet (3.1 m) was adequate to safely carry the building code-specified minimum load. Square-shaped slabs were more susceptible to failure than rectangular-shaped ones. Additional information about the experimental work may be found in the literature (Ling et al., 2023), which is currently under review with the Journal of Coastal Research.

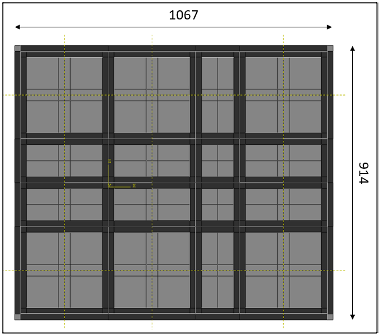
Tool Development
Based on the numerical modeling results, a Home Elevation Decision Tool was developed in 2022 by Nur Yazdani with assistance from Cedric Ling, Debashish Kar, Maria Koliou, and Yoo Yong as a software interface. It allows users to determine the floor load capacity of an elevated home slab rapidly and conveniently, based on the governing building code provisions. The user must log in or create a new account to access the full data set of features, or they may proceed as a guest to use a more limited portion of what the application offers. A disclaimer and a user manual are also available from the home screen (see Figure 3). The Excel-based tool is freely available on Android, iOS, and desktop platforms. It may be found by searching App stores for “Home Elevation Decision Tool.”

The user selects a home model from five available archetypes that most closely resemble the actual home being considered. The selection is tied to the home floor area, number of stories, and roof configuration. The pier spacings in the two directions are then selected, determining the total number of piers in the configuration (see Figure 4).
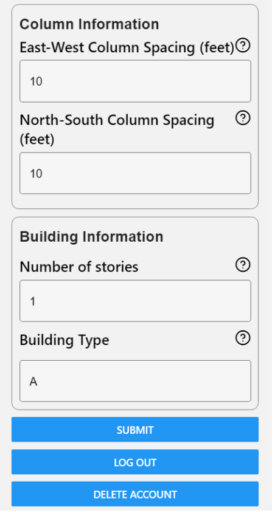
When prompted via the “submit” link, the tool compares the entered selections with a hidden internal database of numerical values. It then outputs whether the specific support beam-pier configuration can safely support the minimum floor loading. A sample results screen from the application is shown in Figure 5, showing the output when the entire elevated slab can safely support the minimum IRC live load.
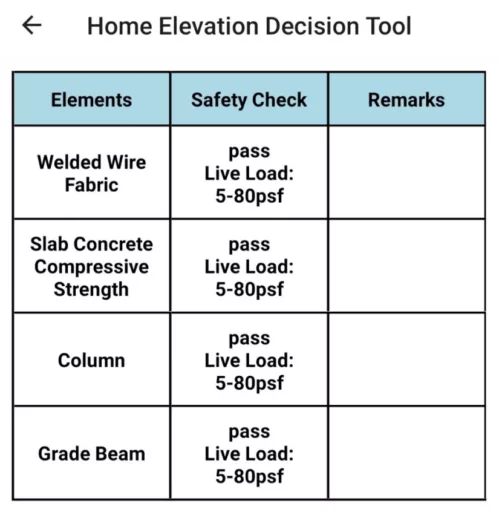
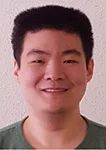
Cedric Ling
-
Cedric Linghttps://www.domprep.com/author/cedric-ling
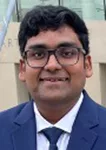
Debashish Kar
-
Debashish Karhttps://www.domprep.com/author/debashish-kar
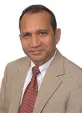
Nur Yazdani
-
Nur Yazdanihttps://www.domprep.com/author/nur-yazdani

Eyosias Beneberu
-
Eyosias Beneberuhttps://www.domprep.com/author/eyosias-beneberu
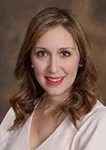
Maria Koliou
-
Maria Koliouhttps://www.domprep.com/author/maria-koliou
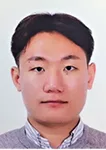
Yong Yoo
-
Yong Yoohttps://www.domprep.com/author/yong-yoo